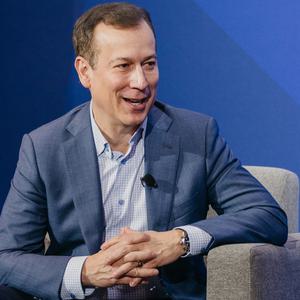
Tessera Therapeutics
In contrast to CRISPR, which evolved to destroy DNA, the machinery to write DNA is the most abundant category of genetic machinery in nature: mobile genetic elements. By engineering and creating synthetic mobile genetic elements, we can move beyond the limitations of gene therapy and gene editing, and pioneer the future of curative genetic medicines: Gene Writing™.
Our genome is a mosaic that has evolved over millennia. It is composed of base pairs, the nucleobases A, C, T, and G—the building blocks of DNA. These represent the code of life and give rise to the complex phenomena that define health and disease. Specific sequences of base pairs encode genes, which serve every function in our bodies. Development, homeostasis, and reproduction; aging, disease, and death—all are driven by the activity of genes.
Doctors know of about 4,000 genes that directly cause diseases. These include the cystic fibrosis transmembrane receptor, which when mutated causes cystic fibrosis, which can severely damage the lungs and other organs to the point of being life-threatening. About 60,000 other genetic sites are significantly associated with traits and diseases such as body mass, cardiovascular disease, neurological conditions, metabolic diseases, immune responses, and many cancers. Every year the number of human genomes that have been sequenced increases, as does our understanding of how genes provide the instructions to make us healthy or unhealthy human beings.
Recently, scientists and doctors have gained the power to add and subtract tiles from our genomic mosaic. This has opened the possibility of creating therapies that not only treat previously untreatable disease but might also cure patients for life with a single treatment. Today, this area, sometimes called genetic medicine, stands upon two technological pillars: gene therapy and gene editing. Both technologies are enormously promising, and in some cases they have already realized their promise and cured diseases. But gene therapy and gene editing have significant limitations in the modifications they can make to the genome, the cells they can affect, and how they are manufactured and delivered to cells, leaving today’s genetic medicine powerless against thousands of diseases.
Origins
In 2017, Flagship partner Geoffrey von Maltzahn, principal Jacob Rubens, associate Rob Citorik, and others began an exploration inside Flagship Labs to address these limitations by asking, What if nature evolved a better way to alter genomes than cutting DNA? The result is Tessera Therapeutics. The company is pioneering a new category of genome engineering technology called Gene Writing and establishing a new field of genetic medicine. Gene Writing writes curative therapeutic messages into the genome. It can specifically and efficiently direct diverse alterations to the genome, in any type of cell, creating new possibilities for patients with genetic diseases.
Gene therapy, the more advanced of the two pillars of contemporary genetic medicine, uses engineered viruses to add DNA to cells in order to treat diseases caused by recessive mutations. Such mutations typically prevent the gene from functioning as it should.
Gene therapy can be divided into ex vivo and in vivo approaches. Retroviral vectors, like the lentiviral vectors derived from HIV, integrate DNA semi-randomly into the genome. They have been the workhorses for ex vivo gene therapy, including the use of engineered immune cells to treat cancer, known as CAR-T cell therapies. Building upon work from Luigi Naldini and others at the San Rafaele Telethon Institute for Gene Therapy, in Milan, GlaxoSmithKline used retroviral vectors to develop Strimvelis, the world’s first ex vivo curative gene therapy (later spun out into Orchard Therapeutics). It has been approved by the European Medical Association to treat a mutation that causes an immune disorder known as bubble boy disease.
For in vivo applications of gene therapy, adeno-associated viruses (AAVs) have emerged as the primary means of delivering DNA into cells. An AAV is a viral vector that delivers episomal DNA (DNA maintained separately from the rest of our genome) to cells. The first gene therapy to be approved in the United States was an AAV therapy developed by Spark Therapeutics (later acquired by Roche) that delivers a gene into the eye to treat a mutation causing impaired vision. More recently, Avexis (acquired by Novartis) developed an AAV gene therapy called Zolgensma, which has enabled children born with spinal muscular atrophy to survive what had been a lethal disease. More than 175 AAV gene therapies are in clinical development at pharma companies such as Pfizer and Roche, large biotechs like Sarepta and Uniqure, and startups like 4D Molecular Therapeutics and Homology Medicine. However, AAV gene therapy is unable to deliver long stretches of DNA and therefore cannot deliver all genes; and it can’t treat diseases that affect dividing cells, because the AAV’s episomal DNA is left behind when the cells replicate. What’s more, because the human immune system attacks and develops resistance to viruses, a gene therapy can only be administered once to any patient, so doctors are unable to adjust the treatment or readminister it if its effect fades.
Gene editing, the second pillar of existing genetic medicine, uses nucleases to cut the genome at a specific location and relies upon cellular DNA damage-response pathways to fix the genetic lesion. The gene editing technology CRISPR, developed by Jennifer Doudna at the University of California, Berkeley; Feng Zhang at the Broad Institute of MIT and Harvard; and others, is being further developed by companies including Editas, CRISPR, and Intellia. CRISPR is remarkably programmable and efficient, but it is only the latest nuclease technology, following upon zinc-finger nucleases, developed by Sangamo; TALENs, developed by Cellectis; meganucleases, developed by Precision Biosciences; and megaTALs, developed by Pregenen and now Bluebird.
These technologies have different features and bugs, but the one manipulation they all do well is cut the genome. That is what the versions that exist in nature evolved to do. CRISPR technology, for instance, is based on a natural defense system that bacteria use to destroy the DNA of invading viruses. By cutting the genome, gene editing can stimulate the process of integrating new DNA into the genome. But this process is inefficient in most cells, because it relies upon DNA damage-response pathways, which are unreliably expressed by the cell and typically repress large genetic alterations.
As a consequence, most gene editing drugs in development are intended to treat diseases that can be addressed by breaking genes without introducing new DNA. These diseases are typically caused by dominant negative mutations, such as the CEP290 mutation that causes Leber congenital amaurosis 10, for which it is better to break the mutated gene than to let it function. Notable exceptions are CAR-T cell therapies and cell therapies for sickle cell disease. The gene editing drugs in development target just 30 nucleotides out of the more than 3 billion nucleotides in our genome. (That’s 0.000001% of the genome!)
With more than 500 ongoing clinical trials, gene therapy and gene editing promise an era in which some of humanity’s most devastating genetic diseases will be cured. But gene therapy cannot complement recessive mutations in long genes or in dividing cells. And gene editing cannot fix dominant mutations in genes whose proper functioning is necessary for health. Further, the viral vectors necessary in gene therapy are inefficient to make, which amounts to a significant impediment to realizing the potential of these technologies.
Breakthrough
Tessera discovered a solution to these problems by harnessing evolution’s greatest genomic architect: mobile genetic elements. Canonically, evolution alters the prevalence of genomes in a population. However, we now recognize that evolution may also act at the level of genes. This theory was popularized by Richard Dawkins’s The Selfish Gene (Oxford University Press, 1976), which argued that our genomes are not only unitary evolutionary entities but are also like villages, composed of thousands of inhabitants called genes, each vying for its own survival. An important piece of evidence for this theory is that some genes evolved the ability to replicate independently of the rest of the genome. These genes are known as mobile genetic elements.
Mobile genetic elements (MGEs) code for the machinery sufficient to move or copy their own DNA into a new location in the genome, and they have been selected over billions of years for their ability to replicate. Barbara McClintock, born in 1902, was a pioneering 20th-century geneticist who unraveled the secrets of genetic recombination and regulation, and she won the 1983 Nobel Prize for her discovery of the first MGE and its mechanism of transposition. She studied the movement of MGEs in corn and demonstrated transposition in a series of classic experiments.
What may have initially seemed like an obscure property of maize’s genome turned out to be a unifying feature of all modern life forms: MGEs are the most abundant genes in the world. There is more DNA in nature that codes for MGEs in natural genomes than there is DNA that does anything else. MGEs are found across the entire animal kingdom, and they also make up a large percentage of the genomes of archaea, bacteria, and plants. In fact, by most measures MGEs make up about 50% of our own DNA, meaning that 1.5 billion nucleotides of our genome code for mobile elements. To put that in perspective, the protein coding genes that we think of as the workhorses of life make up only 2–3% of our genome.
Advantage
Tessera’s founding team saw the opportunity to pioneer a new category of genome engineering technology based upon the rich molecular biology of MGEs. MGEs replicate through DNA and RNA intermediates. The intermediate can be linear or circular, double- or single-stranded; MGEs can transpose, retrotranspose, and recombine; copy and paste or cut and paste; and integrate specifically into certain DNA sequences, semi-randomly into genomic regions, or randomly into any location in the genome. Perhaps most interestingly, MGEs efficiently alter genomes with minimal reliance upon (indeed, often in spite of) other genes in the cell.
Focus
By harnessing and engineering this biology, Gene Writing can break the rules that have governed genetic medicine for two decades. Tessera has identified tens of thousands of MGEs from across the tree of life that are suitable for Gene Writing. Currently, we are developing both RNA-templated and DNA-templated Gene Writing technologies based on engineered and synthetic MGE. RNA Gene Writers can write into or rewrite the genome based upon an RNA template that coordinates base pair changes, small insertions and deletions, or the integration of entire genes. DNA Gene Writers use a DNA template to write large payloads into the genome. With these technologies, for the first time it may be possible to make diverse alterations to the genome, both small and large, without breaking the genome or relying on DNA repair pathways; to deliver RNA to cells in order to add new DNA into the genome without relying on viruses that cannot be administered more than once; and to scalably and affordably manufacture medicines so that cures can reach all the patients in need.
Tessera believes that Gene Writing will become a new field of genetic medicine by making treatments possible for the genetic defects at the root of thousands of diseases. DNA is the code of life—and thus the code that drives an enormous variety of human diseases. Tessera aims to cure rare genetic diseases that gene therapy and gene editing cannot address and to revolutionize medicine by transforming the standard of care for many diseases from treatments to cures. Like artists changing individual tiles—known as tessera—of a mosaic to alter the whole, Gene Writing scientists will change the fundamental unit of biology: genes.
Latest News from Tessera Therapeutics
- Michael Severino, M.D., Joins Flagship Pioneering-Founded Tessera Therapeutics as Chief Executive Officer 06.02.2022
- Tessera Therapeutics Announces Over $300M Series C Financing to Advance its GENE WRITING Platform 04.19.2022
- Flagship Pioneering Expands Biotech Footprint to Property in Somerville’s Boynton Yards 03.31.2021
- Flagship Pioneering’s Scientists Invent a New Category of Genome Engineering Technology: Gene Writing 07.07.2020